Brains in Space: The Impact of Microgravity and Cosmic Radiation on the Brain
- Iván Linares-García
- Apr 21
- 4 min read
About 70 percent of astronauts develop Spaceflight‑Associated Neuro‑Ocular Syndrome (SANS). Our brains evolved under Earth’s gravity and aren’t designed for the “weightless, ion‑filled void” of space. With missions to the Moon and Mars on the horizon, it’s crucial to understand how these extreme conditions affect the brain—and what we can do to protect it.
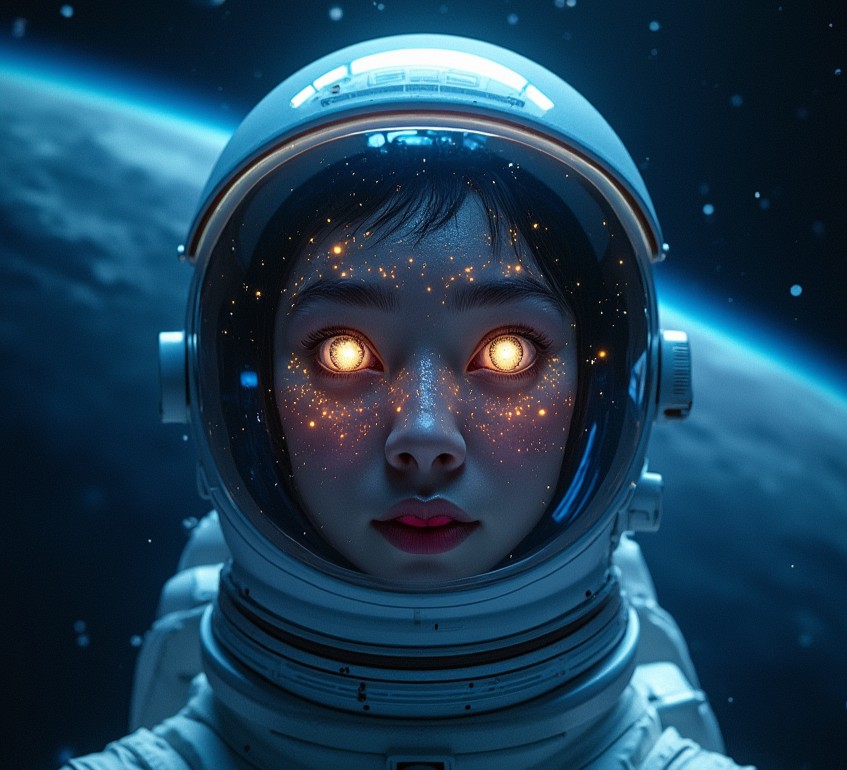
A recent review pulled together findings from many studies on how space travel impacts the nervous system. In the coming years, dozens of crewed missions to lunar orbit and even Mars will expose astronauts to prolonged microgravity and cosmic radiation. That makes it urgent to unravel their effects on the brain over months—or years—in flight.
In NASA’s famous twin‑study, one astronaut spent a year aboard the ISS while his identical twin stayed on Earth. The space‑traveler showed measurable cognitive declines compared to his brother, underscoring that mission success depends not only on cutting‑edge engineering but on optimal brain health. Minimizing these adverse effects will be key to future exploration.
Microgravity and Its Effects on the Brain
You don’t have to leave Earth to taste microgravity—brief free‑fall on a roller‑coaster, diving into a river, or floating in a pool gives you a momentary thrill. But those seconds pale in comparison to the continuous weightlessness of space. Imagine living like that for months or years.
To study these effects, researchers combine data from actual missions with Earth‑based microgravity simulations. In weightlessness, body fluids shift upward toward the head. Cerebrospinal fluid can expand the brain’s ventricles by up to 25 percent, raising intracranial pressure and inflaming the optic nerve—hallmarks of SANS.
The otolith organs in our inner ears, which normally sense head position, become ineffective, destabilizing the vestibular system and making movement awkward. To compensate, the brain increases gray‑matter volume in somatosensory and motor regions—a change that, fortunately, appears to reverse after return to Earth.
Rewiring for Three Dimensions
Spaceflight forces you to navigate a fully three‑dimensional environment, reshaping how the brain maps space. MRI studies have found reduced connectivity between the superior occipital gyrus and the rest of the brain, as well as between the left middle occipital gyrus and areas like the parahippocampal gyrus, cerebellum, and lateral occipital cortex. At the same time, visuomotor circuits grow stronger. Changes in the left caudate nucleus—and an increase in left lateral‑ventricle volume—have been linked to postural control and motor performance.
After landing, sensorimotor readaptation varies with genetics and behavior. Current countermeasures target muscle and bone atrophy, but protocols must also address brain changes. Proposed solutions range from lower‑body negative‑pressure suits and short‑arm centrifuges (artificial gravity) to antioxidants, vitamins, cognitive training, augmented‑reality tools, and specialized rehabilitation.
Cosmic Radiation: The Invisible Threat
On Earth, our atmosphere shields us from the sun’s most dangerous particles. In space, astronauts encounter a cocktail of high‑energy protons, helium nuclei, and heavy ions (HZE)—the latter deemed by NASA a critical health risk. On a three‑year Mars mission, every cell would be traversed by protons roughly every three days, by helium ions every three weeks, and by HZE particles at least twice within that same period.
Rodent studies show that HZE exposure damages DNA, kills neurons, sparks neuroinflammation, depletes neural progenitors, reduces dendritic branching, and compromises the blood–brain barrier—leading to memory and learning deficits. Female rodents often fare better than males, while older animals are more susceptible.
Mitigation Strategies Under Way
To safeguard the central nervous system, NASA’s Combined Behavioral Stressors (CBS) project is defining key biomarkers—molecular, behavioral, and imaging—to monitor brain health in real time. For radiation, researchers are developing advanced high‑density shielding materials and testing neuroprotective drugs (e.g., antioxidants, oxidative‑stress modulators). Specialized resistive‑exercise routines help redistribute fluids and preserve sensorimotor function. Portable EEG and fNIRS devices, now validated for microgravity, allow continuous neural monitoring, and early studies hint that anti‑inflammatory compounds could add another layer of defense.
Looking to the Future
Next steps include experiments that subject animal models to both microgravity and radiation simultaneously—and bringing non‑human primates into the mix for closer human relevance. These efforts will be vital not only for Mars-bound crews but also for the burgeoning space‑tourism industry, where civilians will face similar hazards. Conquering these challenges will deepen our understanding of brain plasticity and ensure the safety—and success—of everyone venturing beyond Earth’s orbit. Our brains, it turns out, are remarkably adaptable—even in realms that once belonged only to science fiction.
Where do body fluids shift in microgravity, and what consequences does this have for the brain?
A. Toward the limbs; it increases muscle fatigue.
B. Toward the head; expands the ventricles.
C. They distribute evenly; there are no noticeable effects.
Key Terms & Definitions
Microgravity An environment of near‑weightlessness experienced in orbit, disrupting fluid balance and sensory signals.
Cosmic Radiation High‑energy particles from space (protons, helium nuclei, HZE ions) that can penetrate tissue and damage DNA.
Spaceflight‑Associated Neuro‑Ocular Syndrome (SANS) A cluster of eye and vision changes—including optic disc swelling and retinal folds—seen in ~70 percent of astronauts on long missions.
Cerebrospinal Fluid (CSF) The protective fluid surrounding the brain and spinal cord; fluid shifts upward in microgravity can raise intracranial pressure.
Otoliths Calcium carbonate crystals in the inner ear that detect head movement; they become less effective in microgravity, causing balance issues.
Vestibular System Inner‑ear structures responsible for balance and spatial orientation; disrupted by weightlessness.
Gray Matter Brain tissue composed mainly of neuron cell bodies; volume changes indicate structural adaptations.
Neural Connectivity The strength of communication between different brain regions; alterations reflect network reorganization.
Neuroinflammation Brain’s immune response, involving microglial activation and inflammatory mediators, which can lead to neuronal damage.
Blood–Brain Barrier A cellular shield that protects the brain from harmful substances; microgravity and radiation can compromise its integrity.
Biomarkers Measurable indicators—molecular, behavioral, or imaging—that signal brain health or dysfunction.
EEG (Electroencephalography) Records electrical brain activity via scalp electrodes; portable versions are now flight‑tested.
fNIRS (Functional Near‑Infrared Spectroscopy) Measures cerebral blood flow changes with infrared light; lightweight and recently used on the ISS.
Reference:
Wuyts, F.L., Deblieck, C., Vandevoorde, C. et al. Brains in space: impact of microgravity and cosmic radiation on the CNS during space exploration. Nat. Rev. Neurosci. (2025). https://doi.org/10.1038/s41583-025-00923-4
Comments